Electroculture is an innovative agricultural method that employs electrical currents or electromagnetic fields to enhance plant growth, health, and yield. By integrating physics and biology, this technique has emerged as a potentially transformative solution to the challenges of sustainable farming. Its origins date back to the 18th century, but advances in technology and ecological awareness have rekindled interest in its application for modern agriculture. Electroculture is seen as a bridge between scientific innovation and environmentally conscious practices.
The fundamental principle of electroculture involves stimulating the natural electrical activity within plants and soil. Plants inherently generate bioelectric signals as part of their growth process. Electroculture techniques amplify these signals, resulting in more efficient nutrient uptake, accelerated photosynthesis, and heightened resistance to environmental stressors such as drought or pests. This method offers multiple benefits without involving chemical additives, making it a clean and renewable alternative.
Various devices and configurations are utilized in electroculture. These include copper coils, antennas, and magnetic fields that channel natural atmospheric energy toward crops. The technology is versatile and scalable, allowing implementation in diverse farming systems ranging from large-scale agricultural operations to small urban gardens.
Research on electroculture has shown promising results. Studies indicate its capacity to reduce the need for synthetic fertilizers and pesticides while improving soil fertility over time. Farmers adopting this technique have reported increased harvests, improved crop quality, and cost savings. As the global demand for sustainable food production rises, the potential of electroculture continues to gain recognition, paving the way for further exploration and adoption.
The Science Behind Electroculture: Understanding How it Works
Electroculture operates on the principle of harnessing natural energy fields to influence plant growth and soil health. This innovative agricultural technique employs electrical currents, magnetic fields, or both to stimulate biological and chemical processes within the soil and plants. While the concept has roots in 18th-century experiments, advancements in science and technology have made its application more precise and efficient in recent decades. At its core, electroculture leverages energy phenomena to enhance the natural processes that support plant vitality.
When low-frequency electromagnetic fields are applied to soil or plants, they interact with ionized particles in the surrounding environment. This interaction can enhance ion mobility, improving the availability of essential nutrients like nitrogen, phosphorus, and potassium to plant roots. Additionally, electrical stimulation may promote root elongation and cell division, leading to accelerated growth and more robust root systems. These effects create an environment conducive to increased productivity and resilience.
Electroculture also impacts microbial ecosystems within the soil. Positive or negative electrical charges influence microorganisms that play a critical role in nutrient cycling and organic matter decomposition. By fine-tuning the electrical environment, farmers can foster beneficial microbial communities while suppressing harmful pathogens. This enables healthier soil with reduced reliance on chemical fertilizers or pesticides.
Researchers have suggested that the electrical effects extend to water molecules retained in the soil. Changes in the polarity of these molecules may enhance soil hydration and nutrient absorption, particularly in regions suffering from drought or soil salinity. Furthermore, plants exposed to electroculture often exhibit improved resistance to environmental stressors such as pests, temperature extremes, and diseases.
The science of electroculture is supported by ongoing experiments measuring electrical properties in agriculture. These studies aim to refine the understanding of field applications and optimize the use of renewable energy sources like solar or wind to power electroculture devices, making the technology even more sustainable.
Historical Roots: The Early Experiments with Electroculture
The concept of electroculture can be traced back to the late 18th and early 19th centuries, when scientists and agriculturalists began exploring the relationship between electrical forces and plant growth. Early experiments sought to determine whether electricity, a burgeoning field of study at the time, could offer practical applications for enhancing crop production. These pioneering efforts laid the groundwork for modern electroculture practices.
One of the earliest documented instances comes from the work of Abbot Nollet in the 1740s, who observed that electrical discharges could stimulate the germination of seeds. His findings sparked curiosity among scientists, who began conducting controlled experiments to investigate this phenomenon further. Fast forward to the 19th century, researchers such as Hugo von Mohl and J.S. Stone amplified interest by testing the effects of atmospheric electricity on plants. Observations revealed that certain types of electrical stimulation appeared to accelerate growth rates or improve plant vitality.
By the late 1800s, commercial interest grew, resulting in the development of rudimentary electroculture apparatuses. Devices like electrified rods for soil and copper wiring grids were implemented to distribute low-level electrical currents to fields. Proponents of these technologies argued that electricity improved ion exchange within the soil and boosted nutrient uptake in plant roots. The experiments were performed alongside the agricultural revolution of the era, offering an alternative to chemical fertilizers.
Though skepticism from some sections of the scientific community limited widespread adoption of electroculture, these early experiments undeniably opened new avenues for innovation. As the 20th century approached, interest shifted due to advances in synthetic agricultural inputs, but the foundations of electroculture as a theoretically sustainable practice persisted.
Why 2025 is a Pivotal Year for Electroculture Adoption
2025 stands at the crossroads of innovation and necessity in agriculture, marking a decisive moment for the adoption of electroculture. Several converging factors are creating an opportune environment for this sustainable and energy-efficient farming method, making it increasingly viable on a global scale.
Policy Shifts and Regulatory Support
Many governments are introducing policies aimed at combating climate change and boosting sustainable farming techniques. By 2025, subsidies and grants for eco-friendly agricultural methods, including electroculture, are expected to expand significantly. Policy frameworks are aligning to incentivize the use of low-impact technologies, making it more accessible for smallholders and large-scale farmers alike.
Technological Advancements
Innovations in renewable energy and hardware technologies are making electroculture tools more efficient and affordable. Lighter, more portable, and durable systems are becoming available, enabling widespread adoption even in developing regions. Improved solar-powered devices and energy storage solutions are reducing dependency on traditional grids, which is particularly beneficial for remote farming operations.
Electroculture technologies, which once seemed niche, are becoming commercially scalable thanks to these advancements.
Rising Economic Pressures
Global food security concerns are intensifying due to inflation, resource scarcity, and erratic weather patterns. These challenges are forcing farmers to seek cost-effective solutions to enhance yields without over-reliance on chemical inputs. Electroculture offers a viable way to reduce input costs while maintaining soil health, aligning with these economic demands.
Heightened Awareness and Education
Public and industry awareness about electroculture is growing, supported by research, media coverage, and academic institutions. By 2025, numerous studies are expected to validate electroculture’s benefits, helping to dispel skepticism and bolster its credibility within the agricultural sector. Training programs and knowledge-sharing platforms are equipping farmers with the expertise needed to implement the technique efficiently.
These intersecting trends make 2025 a transformative year for integrating electroculture into mainstream farming practices, setting the stage for a more sustainable agricultural future.
Key Benefits of Electroculture
Electroculture, a centuries-old agricultural innovation, has emerged as a transformative tool for modern farming practices. By leveraging electrical currents or magnetic fields to enhance plant growth, this method offers a range of significant advantages for producers and the environment alike.
Increased Agricultural Yields
One of the primary benefits of electroculture is its ability to enhance crop yields. Studies indicate that plants exposed to subtle electrical stimulation exhibit accelerated growth rates, improved nutrient absorption, and an increase in biomass. This results in higher quantities of produce per harvest, addressing rising global food demands while empowering farmers to maximize their land’s output.
Reduced Dependence on Chemical Inputs
Electroculture minimizes reliance on chemical fertilizers, pesticides, and herbicides by boosting the inherent vitality and resilience of plants. This natural enhancement of plant health diminishes the need for synthetic solutions, reducing costs for farmers and lowering the risks of soil and water contamination.
Enhanced Soil Health
The use of electroculture has shown promise in reversing the degradation of soil properties. It strengthens the microbial activity and nutrient availability in soil, contributing to a healthier, more balanced ecosystem. This, in turn, promotes sustainable farming practices and greater fertility over time.
Energy Efficiency and Cost Savings
In comparison to conventional agricultural technologies, electroculture often requires minimal energy input. Devices used in the process operate efficiently, contributing to reduced operational costs. Furthermore, its low-maintenance nature makes it accessible for small-scale farmers and large enterprises alike.
Environmental Benefits
Electroculture solutions align seamlessly with climate-smart agriculture initiatives. By reducing chemical runoff, conserving soil integrity, and lowering carbon footprints associated with synthetic inputs, this approach underscores its potential to mitigate agriculture’s environmental impact and support long-term ecological health.
Through its multifaceted benefits, electroculture positions itself as a crucial component of a sustainable agricultural future.
How to Get Started with Electroculture: Tools and Techniques for Beginners
Electroculture, a growing agricultural practice utilizing natural electrical currents to enhance plant growth, offers numerous benefits for sustainable farming. Beginners keen on exploring this method can lay the groundwork by understanding the essential tools and techniques necessary for success.
Basic Tools for Electroculture
A successful introduction to electroculture begins with assembling the appropriate tools. Essential items include:
- Copper wire: As a key conductor, copper wire is used to harness and channel atmospheric electricity to plants.
- Wooden stakes or rods: These function as grounding supports for the wire and are often crafted from untreated wood to maintain natural conductivity.
- Magnets (optional): Depending on the technique, ferrite or neodymium magnets can be used to influence magnetic fields around crops.
- Wire cutters and pliers: Basic tools for shaping and attaching wires to stakes are necessary.
- Insulated gloves: For safe handling of wires during setup and adjustments.
Beginner-Friendly Techniques
A step-by-step approach ensures effective use of electroculture methods:
- Install antennas: Wrap copper wire around wooden stakes, spiral it upwards, and place the stakes near plants. The wire collects atmospheric charges to stimulate soil and plant health.
- Grounding the wire: Insert the stake deeply into the soil to establish a direct connection with Earth’s natural energy field.
- Air ionization techniques: Install wire spirals at varying heights to attract air ions beneficial to plant growth.
- Magnet integration: Position magnets near the root zone to enhance nutrient absorption and boost metabolic activity in plants.
Monitoring Progress
For beginners, maintaining a consistent observation routine can be critical. Tracking plant growth, soil health, and environmental conditions will help refine techniques over time. Regular adjustments to wire configurations and magnet placements can further optimize results.
Success Stories: Farmers Who Transformed Their Crops with Electroculture
Over recent years, electroculture has emerged as a breakthrough strategy for revitalizing agricultural yields, and numerous farmers worldwide have reported remarkable transformations. These success stories highlight the potential of this technique when paired with innovative thinking and sustainable practices.
One example comes from a French vineyard owner who employed a basic electroculture setup using copper antennas to capture and distribute atmospheric electricity within the soil. The results were extraordinary. The vineyard saw a 25% increase in grape yield, enhanced sweetness in the fruit, and stronger resistance to common fungal diseases. This farmer noted significant savings in chemical fungicides, further reducing environmental impact.
Meanwhile, a rice farmer in Japan adopted electroculture to address previously declining harvests. By embedding low-cost conductive coils into waterlogged paddy fields, he achieved notably taller, healthier plants. Additionally, his crops matured faster, improving growing cycles and allowing for an extra harvest within the same year. The farmer stated that the improved aeration and microbe activity in the soil were major contributors to his success.
In Kenya, a smallholder farmer incorporated solar-powered electroculture devices into her vegetable fields. With minimal investment, she experienced increased production of staple crops such as tomatoes and spinach. Her farm also saw a reduced need for irrigation and fertilizers, a critical advantage in an arid region where water scarcity is commonplace.
These stories underline the versatility of electroculture, proving its effectiveness across climates and crop types. Farmers consistently emphasize how its application not only boosts productivity but also supports resilience against climate-related challenges.
Comparing Electroculture with Traditional Farming Methods
Electroculture, an innovative agricultural practice, integrates electromagnetic fields and electrical currents to stimulate plant growth and improve soil conditions. Traditional farming methods, in contrast, rely on mechanical tools, chemical fertilizers, pesticides, and manual labor to achieve crop production goals. Examining the differences between these approaches reveals insights into their efficiency, sustainability, and long-term impacts on the environment.
One of the primary distinctions lies in resource consumption. Electroculture utilizes minimal external inputs like synthetic chemicals and water, leveraging natural electric currents to enhance plant metabolism. Traditional farming often depends heavily on chemical fertilizers and pesticides, leading to concerns about soil degradation, water contamination, and resistance in pests. Electroculture addresses these environmental challenges by promoting natural growth processes, reducing ecological strain.
Labor and energy efficiency also set these methods apart. Traditional farming can be labor-intensive, with significant energy demands for irrigation, tilling, and pest control. Conversely, electroculture employs passive systems, such as copper antennas or scalar technology, which require little maintenance and operate sustainably over time. This optimizes productivity while decreasing reliance on fossil fuels.
Soil health management further highlights the divergence between the two. Traditional methods can disrupt soil microbial life through repeated tillage and chemical usage. Electroculture, by contrast, supports healthier microbial ecosystems through gentle stimulation of soil with electrical fields. Enhanced aeration and nutrient availability foster resilience and biodiversity in farming systems.
Economic considerations remain a key area of comparison. Traditional farming often incurs ongoing costs for chemical treatments and energy. Electroculture, while requiring an initial investment in infrastructure, reduces operational expenses over time by lowering input requirements.
The performance of both methods against climate change adaptability underscores their divergence. Electroculture techniques have the potential to mitigate climate risks by increasing drought resistance and crop yields, while traditional methods face limitations in unpredictable weather patterns. These differences continue to reshape discussions around sustainable agriculture in the modern era.
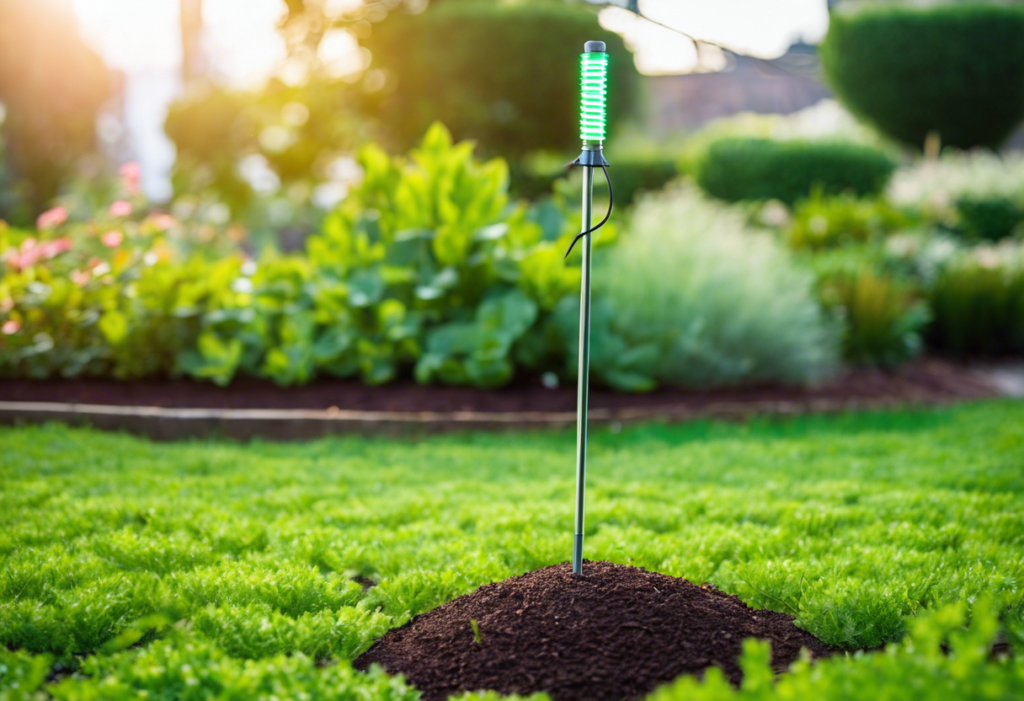
Challenges and Misconceptions About Electroculture
Electroculture, while promising significant advancements in sustainable agriculture, faces multiple challenges and pervasive misconceptions. These barriers often stem from gaps in research, integration difficulties, and misinformation.
Challenges in Implementation
- Limited Scientific Validation: Although studies and anecdotal evidence suggest positive effects of electroculture, extensive peer-reviewed research validating its scalable impact remains scarce. This lack of rigorous validation often deters stakeholders from investing in its adoption.
- Infrastructure and Cost Barriers: Setting up systems for electroculture—such as conductive coils, antennas, or energy sources—may require technical expertise and financial investment. Small-scale farmers, particularly in less developed regions, often face challenges in acquiring necessary resources.
- Regulatory and Policy Gaps: Governments worldwide have been slow to recognize electroculture as a viable agricultural practice. The absence of regulatory frameworks supporting subsidies, technical training, or widespread adoption hinders momentum.
- Adaption to Soil and Climate Conditions: Electroculture’s effectiveness is influenced by regional variables such as soil composition, precipitation, and climate. Inconsistent results across locations can discourage its implementation.
- Resistance to Innovation: Long-standing agricultural practices often resist change. Farmers who are unfamiliar with electroculture may view it as unscientific or impractical.
Pervasive Misconceptions
- Electroculture is often mistakenly perceived as pseudoscientific or reliant purely on mystical explanations rather than grounded in electromagnetic principles. Miscommunication about the method’s scientific basis weakens its credibility.
- There is a belief that electroculture eliminates the need for fertilizers or traditional farming techniques altogether. This oversimplification creates unrealistic expectations about its capabilities.
- Concerns about environmental harm arise from misconceptions linking electroculture to energy-intensive, industrial applications. Many assume that it heavily relies on external energy sources rather than harnessing renewable or passive environmental electricity.
A combination of scientific outreach, education, and research is key to overcoming these barriers and addressing prevalent misunderstandings. Misinformation and practical hurdles must be tackled systematically to unlock electroculture’s transformative potential.
The Role of Technology in Shaping Electroculture’s Future
Advancements in technology are playing a transformative role in redefining the capabilities and scalability of electroculture in agriculture. At the core of this progression is the integration of modern digital tools and innovative hardware, which are unlocking new dimensions of efficiency, precision, and accessibility.
The development of Internet of Things (IoT) devices has enabled real-time monitoring and control of electroculture systems. Through IoT sensors, farmers can gather crucial data about soil conductivity, moisture levels, and atmospheric conditions. This granular data allows for precise calibration of electromagnetic outputs, ensuring crops receive optimized stimulation based on environmental factors. Machine learning algorithms further enhance this process by analyzing collected data to recommend adaptive usage patterns tailored to specific crop needs.
Renewable energy technologies are also revolutionizing electroculture implementation. Solar panels and wind turbines are now being integrated with electroculture setups, providing a sustainable and cost-effective energy source for powering electromagnetic devices. This innovation reduces dependency on conventional energy grids, making electroculture more viable for rural and off-grid farming communities.
Another critical advancement lies in the miniaturization and cost reduction of electroculture hardware. Compact coils, electrodes, and capacitors are being designed to lower operational costs while maintaining output efficacy. These designs are making electroculture accessible to small-scale farmers who may lack large capital investments.
Blockchain technology is streamlining operations across the supply chain by enabling transparent tracking of crop production metrics. Farmers utilizing electroculture can document and verify claims about yield increases or reduced chemical usage, which can appeal to environmentally-conscious consumers and regulatory bodies alike.
Collaborations between researchers, tech firms, and agricultural institutions are laying the groundwork for widespread innovation. For example, open-source databases are sharing electroculture performance data, fostering community-driven experimentation and progress.
These technological advancements are creating the potential for electroculture to move from niche applications to mainstream farming paradigms in the years to come, broadening its impact on sustainable food production.
Electroculture’s Environmental Impact and Potential for Global Food Security
Electroculture offers a compelling approach to sustainable agriculture, presenting positive environmental implications while addressing pressing concerns surrounding global food security. This innovative method, centered on harnessing atmospheric and electromagnetic energies to stimulate plant growth, has the potential to transform modern farming practices.
One notable environmental benefit of electroculture is its ability to reduce reliance on synthetic fertilizers and pesticides. By optimizing plant nutrient uptake and resilience through natural electromagnetic stimulation, farmers can minimize chemical inputs that often pollute soil and water systems. This shift supports healthier ecosystems, reduces groundwater contamination, and minimizes the carbon footprint associated with chemical production and transportation.
Moreover, electroculture aligns with regenerative agriculture practices by promoting soil health. Enhanced root growth and microbial activity, often observed in electroculture experiments, contribute to improved soil structure and carbon sequestration. This effect not only supports plant productivity but also offers a scalable solution to combating climate change as atmospheric carbon levels continue to rise.
The method’s adaptability across diverse climates and soil conditions positions it as a strategic tool for addressing food insecurity. As global populations grow, particularly in regions facing arid conditions or degraded land, electroculture can provide a low-cost, resource-efficient alternative to conventional farming. This approach encourages higher yields without depleting finite natural resources, offering greater resilience against extreme weather caused by climate change.
Energy efficiency represents an additional asset. Unlike mechanized systems dependent on fossil fuels, electroculture demands minimal energy input, often relying on renewable resources such as solar or atmospheric electricity. This feature makes it particularly attractive for smallholder farmers in underdeveloped regions, where access to conventional energy sources is limited.
Electroculture also fosters biodiversity. By reducing chemical interventions and improving soil health, it encourages populations of beneficial organisms and supports pollinators, both of which play pivotal roles in ensuring long-term agricultural sustainability. This dual focus on ecological integrity and yield enhancement positions electroculture as a viable pathway toward achieving global food security.
Practical Tips for Scaling Electroculture Across Larger Agricultural Systems
Electroculture can significantly enhance productivity when scaled to large agricultural systems. However, its successful implementation requires thorough planning, resource management, and adaptability to varying conditions.
- Assess Field-Specific Requirements A critical first step involves evaluating environmental and soil conditions for each site. Variables such as soil pH, moisture levels, and mineral content influence how electroculture methods interact with the terrain. Conducting detailed surveys ensures that the system is customized to local farming needs and avoids over-generalized solutions.
- Invest in Scalable Infrastructure For large-scale applications, durable and scalable materials, such as conductive wires, antennas, or copper coils, are crucial. These materials should withstand environmental stressors like wind, rain, and temperature fluctuations. Utilizing modular electroculture designs can allow for phased growth, enabling farmers to expand systems incrementally while minimizing costs.
- Optimize Energy Sources Electroculture systems require reliable energy sources, whether these involve solar panels, batteries, or wind turbines. Renewable energy options can reduce operational costs and align with sustainability goals. Energy efficiency audits are necessary to ensure systems run effectively without overconsumption or unnecessary downtime.
- Implement Precision Monitoring Systems Technology integration is essential for managing large-scale electroculture setups. IoT sensors and data analytics can track electrical field intensity, plant growth rates, and nutrient uptake in real time. These tools provide actionable insights, helping farmers adjust their systems dynamically to achieve optimal results.
- Train Personnel Thoroughly Scaling electroculture demands skilled labor capable of managing and troubleshooting sophisticated equipment. Training programs should focus on the technical principles of electroculture, system maintenance, and troubleshooting common issues. This ensures smooth operations and maximized output.
- Adopt Incremental Implementation Rolling out electroculture incrementally can mitigate risks associated with large-scale adoption. Farmers can begin with pilot sections to test efficacy under localized conditions. This allows for the identification of potential challenges before system-wide deployment.
By building strong communication networks within farming communities, practitioners can share data, insights, and methods to foster continuous improvement.
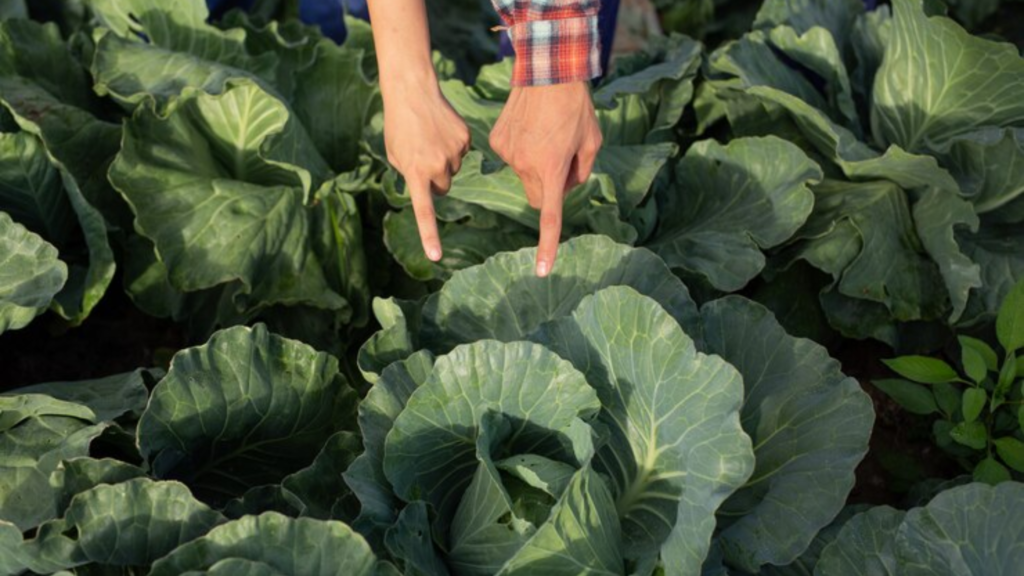
Government Policies and Industry Support for Electroculture Advancements
Governments worldwide are increasingly recognizing electroculture as a potential solution to address the challenges of sustainable agricultural development. Policy frameworks are being developed to encourage its adoption, with a focus on funding, research support, and infrastructure alignment. Many countries are incorporating electroculture technologies into their broader climate and food security strategies to enhance soil health, optimize crop yields, and reduce dependency on chemical fertilizers.
Several agricultural departments and ministries are rolling out subsidies to incentivize farmers to adopt electroculture methods. These subsidies often target smallholder farmers, aiming to lower financial entry barriers for the use of advanced techniques such as electric coils, antennas, and magnetic setups that enhance plant growth. Additionally, national programs are funding pilot projects and field trials to explore how electroculture integrates into diverse farming systems, particularly in areas with depleted soils or poor irrigation facilities.
On the global stage, organizations such as the Food and Agriculture Organization (FAO) are collaborating with member nations to establish clear protocols for electroculture applications. These partnerships are also addressing the need for regulatory frameworks to ensure the safety and efficacy of electromagnetic and electric field applications on crops. International forums are serving as platforms for knowledge sharing, helping countries learn from one another’s success stories.
Industry leaders in agritech are augmenting these efforts by investing heavily in innovation and commercialization. Private firms are partnering with research institutions to refine electroculture tools, making them scalable and accessible for diverse farm sizes. Meanwhile, startups are driving advancements in cost-effective, energy-efficient devices designed for lower-income regions. These collaborations between the public and private sectors are fostering an environment where electroculture innovations can thrive, paving the way for a transformative impact on global agriculture.
Common Questions Answered: Electroculture FAQ
Electroculture, a method of enhancing plant growth by leveraging electrical and magnetic fields, is capturing global attention as sustainable agriculture techniques evolve. Below are answers to frequently asked questions to help demystify this promising practice.
1. What is electroculture, and how does it work?
Electroculture utilizes natural atmospheric energy, such as static electricity or magnetic fields, to stimulate plant growth. Conductors, coils, or specific antenna systems are integrated into the soil or placed near crops. These devices enhance soil ionization and improve nutrient absorption, promoting faster and healthier growth. It is an eco-friendly approach that does not rely on chemical fertilizers or pesticides.
2. Is electroculture technology safe for crops and the environment?
Yes, electroculture is considered environmentally safe and sustainable. It eliminates harmful agricultural inputs like synthetic chemicals while naturally boosting plant health. The method does not generate harmful emissions or residues, preserving biodiversity and soil quality.
3. Does electroculture work in all climates and soil types?
Electroculture has demonstrated efficacy across various climates and soil types. However, results can vary depending on the crop species, the design of the electroculture system implemented, and environmental conditions. Adjustments to the technology may be necessary to optimize outcomes in extreme conditions.
4. What crops benefit the most from electroculture?
Electroculture is versatile, supporting the growth of vegetables, grains, fruits, and even ornamental plants. Crops with high nutrient demands, such as tomatoes or leafy greens, typically show the most significant improvements.
5. How cost-effective is electroculture for modern farmers?
Electroculture systems have a relatively low setup cost compared to traditional agricultural technologies. They require minimal maintenance, and the reduction in expenses for fertilizers and pesticides makes this an attractive long-term investment.
6. Can electroculture replace traditional farming practices entirely?
While electroculture serves as a sustainable supplement, it may not entirely replace traditional methods on large-scale farms—at least in the near term. However, integrating it into existing practices enables farmers to achieve higher yields with fewer environmental consequences.
Conclusion: Embracing a Greener, More Efficient Future in Agriculture
Electroculture represents a transformative approach to agriculture, blending age-old farming practices with modern scientific understanding. By leveraging the Earth’s electromagnetic fields, farmers can reduce dependency on chemical fertilizers and pesticides, enhance soil health, and improve crop yield without compromising the environment. This practice aligns with the global push toward sustainable solutions—a critical step as climate change continues to challenge food systems worldwide.
Key advances in materials science and renewable energy technology have made electroculture tools not only more effective but also economically accessible. The integration of these tools into mainstream agriculture promotes resource efficiency, enabling growers to harness natural forces while reducing costs. For smallholder farmers, particularly in regions with limited access to synthetic inputs, this innovation holds the potential to close productivity gaps, ensuring food security for growing populations.
Additionally, electroculture provides meaningful opportunities to address soil degradation—a pervasive issue in many arable regions. Practices such as magnetic soil conditioning and energy-assisted plant stimulation rejuvenate exhausted soils, restoring fertility and encouraging biodiversity. Coupled with its ability to strengthen plant resilience against pests and extreme weather, electroculture emerges as a practical solution in both developed and developing agricultural systems.
By adopting electroculture, industries can also mitigate their carbon footprint. Reduced chemical input lowers greenhouse gas emissions associated with conventional farming, contributing to a more climate-resilient agricultural future. Transitioning to this sustainable framework requires collaboration across agricultural sectors, research institutions, and government bodies.
The potential for electroculture to redefine modern farming is magnified by its environmental benefits and cost efficiency. With ongoing research and strategic implementation, it has the capacity to bridge the gap between productivity and ecological responsibility, paving the way for a greener, more harmonious relationship between humanity and the Earth.